Research
Article
Voluntary Consumption of
Concentrated Oral Nicotine Solutions and Serum Nicotine
Detection by GC-MS
Bryanna Broadaway, L. Elise Eubank and Amy R. Pearce
Arkansas State University
Address correspondence to Amy R. Pearce, Ph.D, Associate
Professor of Psychology, Adjunct Assistant Professor of Biology,
PO Box 1560, State University, AR 72467, e-mail:
apearce@astate.edu
ABSTRACT:
We extend our earlier work on the influence of environmental
availability of nicotine by increasing the concentrations of
oral nicotine solutions and by using gas chromatography-mass
spectrometry (GC-MS) applications to examine nicotine and its
primary metabolite cotinine in the blood serum. Sprague Dawley
rats were chronically exposed to nicotine solutions (5mg/ml
and 8mg/ml) through drinking bottles in
their home cage. At the end of oral nicotine exposure, blood
serum was analyzed by GC-MS for nicotine and cotinine content.
Intake results supported previous studies showing rats will
readily and voluntarily ingest oral nicotine, even at relatively
high concentrations. Further, GC-MS results indicated nicotine
in the majority of spiked and unspiked samples, but with wide
variations in nicotine serum counts. Although GC techniques need
to be refined, this study is the first to establish that
nicotine concentrations delivered via a truly voluntary oral
route results in pharmacologically detectable levels of the drug
in biological samples and this knowledge advances our
understanding and future application of the oral nicotine model.
Nicotine is the main substance with psychoactive properties found
in tobacco products (Gutkin,
Dehaene, & Changeux, 2006;
Bolliger et al., 2000) that
creates great potential for addiction by altering reward systems and
relevant psychomotor and cognitive processes in the brain (Besson et
al., 2007).
Once ingested, nicotine
breaks down into a number of metabolites, including
cotinine which is the major nicotine
metabolite. Within the human body the half-life of cotinine is
approximately 17 hours, whereas the half-life of nicotine is
only about two hours (Wall, Johnson,
Jacob, & Benowitz, 1998). Cotinine concentration in various body
fluids is considered to be among the most useful markers of nicotine
exposure currently available (Swan, Habina, Means, Jobe, &
Esposito, 1993). Due to the long life of
cotinine in biological samples, it has been used as an indicator of
recent tobacco smoke or nicotine exposure (Sepkovic & Haley, 1985).
Many techniques have been
developed to detect nicotine and/or cotinine in different biological
matrices. Thin layer chromatography
(TLC) has been used for the detection of
nicotine and cotinine in human urine by a solid-liquid extraction
and this technique has been applied in the sampling of urine from
children who were exposed to environmental tobacco smoke (Tyrpien et
al., 2000). TLC techniques have also shown that nicotine is more
efficient than cotinine at passing the blood-brain barrier in rats
by measuring the nicotine and cotinine levels in brain tissue (Riah,
Courriere, Dousset, Todeschi, & Labat, 1998).
Another technique, enzyme-linked
immunosorbent assay
(ELISA) has been used to test a
vaccination against nicotine during continued nicotine
administration in rats and the effects on nicotine distribution to
the brain (Hieda, Keyler, Ennifar, Fattom, & Pentel, 2000).
ELISA was also used to study the detection of nicotine and cotinine
in urine, serum, and saliva of active and passive human smokers (Ziegler,
Kauczok, Dietz, Reith, & Schmidt, 2000;
Langone, Cook, Bjercke, & Lifschitz, 1988; Eramo et al., 2000;
Benkirane, Nicolas, Galteau, & Siest, 1991).
Gas chromatography (GC)
coupled with mass spectrometry (MS) is
often considered the gold standard in substance detection, including
drugs of abuse. This assay method has been employed for the
simultaneous quantification of nicotine and cotinine in urine of
passive and active smokers (Man, Gam, Ismail, Lajis, & Awang, 2006;
Heinrich-Ramm, Wegner, Garde, & Baur, 2002)
and for direct nicotine delivery in nonhumans (Jung, Chung,
Chung, Lee, & Shim, 1999). Several
extraction methods exist to quantify nicotine and cotinine in plasma
or serum by GC-MS using ion trap detection in plasma or serum of
rats and humans (e. g., Cognard & Staub, 2003;
Jacob, Wu, Yu, & Benowitz, 2000; Jung, et al.).
In previous studies, we demonstrated
that nicotine can be administered chronically and voluntarily in the
drinking water of rats and that the environmental availability of
the oral nicotine influences ingestion (Biondolillo & Pearce, 2007;
Boyett, Pearce, & Biondolillo, 2007). Though there is systemic
absorption of pharmacologically significant amounts of nicotine when
delivered orally, this route relies on the relatively slow process
of gastric absorption and first-pass metabolism before becoming
available in the bloodstream. Hence, questions arise concerning both
the speed with which nicotine becomes available and the amount of
nicotine ultimately available to the central nervous system,
important issues for the development of dependence (for a review see
Warnakulasuriya, Sutherland, & Scully, 2005). In this study, we used GC-MS applications to examine nicotine and
cotinine levels in the serum of rats exposed to nicotine through our
oral model.
MATERIALS AND METHODS
Animals
Forty-eight Sprague-Dawley rats (20
male; 28 female: Harlan Company, Indianapolis, Ind., USA) served as
subjects. Rats were approximately 25 days old at the beginning of
the study and housed in sex-matched pairs within clear polycarbonate
cages (Allentown Caging) fitted with Cell-Sorb Plus bedding topped
with stainless steel wire lids. The subjects were on a 12:12 light:
dark cycle with the lights on from 1300 through 0100. While in the
cages, the rats had free access to Purina Lab Diet 5012 and water at
all times. The colony room was maintained at 20-22 oC.
The Arkansas State University Institutional Animal Care and Use
Committee approved all procedures.
Administration of oral nicotine
Centrifuge tubes (50 ml) fitted with
rubber stoppers and stainless steel drinking tubes were used for the
drinking bottles. All rats had six bottles placed on the top of the
wire lids through which they could access the drinking tubes. As a
counter measure, the bottles were presented in varying sequences
atop each cage with all like solutions in consecutive pairs. For all
subjects bottle positions remained constant throughout the study to
increase the likelihood that rats could discriminate between
nicotine solutions and water. Nicotine solutions were made by mixing
nicotine base (Sigma Aldrich) in tap water at concentrations of
5
mg/ml and 8
mg/ml nicotine base/water. Solutions were mixed approximately once a week
and stored in amber bottles. The rats received two bottles of the 5
mg/ml mixture, two bottles of the 8
mg/ml mixture, and two
bottles of tap water. All subjects received the six bottle
arrangement. Each bottle was identified by cage, subject(s), content
and order of presentation on the lid of the home cage. As in
previous studies from our lab (Biondolillo & Pearce, 2007;
Biondolillo, Pearce, Louder, McMickle, under review), bottles were
filled with nicotine solution or water and the mass of intake for
each drinking bottle was measured daily. Approximately 23 hours
later bottles were removed, weighed again, and a difference score
was calculated by subtracting removal bottle weight from placement
weight (g), a procedure considered to be a reliable measure of fluid
intake (Stolerman & Kumar, 1972). This score was used to reflect
intake from individual bottles in the previous 23 hour period. Body
weights were collected every third day. Bedding and food were
changed and replaced once a week. All subjects were exposed to all
liquids for 23 hours out a of 24 hour period. During the 1 hour of
nonexposure intake data were recorded and cages were cleaned. This
arrangement was presented for 42 consecutive days for 6 pairs of
subjects, and 63 consecutive days for 13 pairs of subjects as well
as for 10 subjects that were separated from their home cage partner
and housed individually for 2 weeks at the end of the study.
Considering that all rats had entered the stage of young adulthood
and the age variations did not necessarily reflect different
developmental stages, such as adolescence (Spear, 2000), intake data
were combined during analysis for same-sex rats sacrificed at the
different time points.
The experimental design was
part of a larger study examining chronic intake of oral nicotine in
maternally and nonmaternally exposed rats, the results of which are
not reported here. For this aspect of the study, we were blind to
maternal exposure assignment, female rats were not pregnant and
nicotine intake was examined only during the 23 hour exposure period
immediately previous to termination and blood collection.
Chemicals
Nicotine and cotinine standards and
analytical grade methanol, dichloromethane, petroleum ether and
ethyl ether were purchased from Sigma (St. Louis, MO, USA).
Standards and Controls
Solutions of reference standards were
prepared in methanol and these standards were used to create
calibration curves as a control. Serum standards were prepared by
adding known amounts of the nicotine standard or cotinine standard
to serum of nicotine exposed rats. Referred to as spiked samples,
this approach allowed for matrix matching to unspiked samples.
Instruments and chromatographic
conditions
A Varian CP3800 (Walnut Creek, CA,
USA) gas chromatograph equipped with a Saturn 2200 ion-trap detector
performed the gas chromatographic analyses. Helium was used as the
carrier gas with a head pressure of 5 psi, and the flow rate was
1ml/min. A Restek capillary column of 60mRtxiTM-5ms x
.53 mm ID was used, coated with a 0.25
mm film. Instrument
settings were based on Elobeid’s (2006) initial measurements of
nicotine in tobacco smoke but chromatographic conditions were
modified to suit elution of the compound in serum. The column
temperature was programmed from an initial temperature of 45 oC
held for 2 minutes, increased to 110 oC at 35 oC/min,
then increased to 200 oC at 25oC/min held for
2 min, and finally to 280 oC at 12 oC/min and
held for 3.88 min. The injection port temperature was 250 oC.
Injections were made in splitless mode (1:1, redundant) using the
CP-8400 Varian autosampler. The ion-trap was operated in electron
ionization with methanol as the liquid reagent. The transfer line,
manifold and trap temperatures were 150 oC, 80 oC,
and 250 oC, respectively.
Instrument control and data acquisition were carried out
using the Varian Workspace. For quantification NICO m/z 84 and COT
m/z 98 were used. The complete identifications for nicotine and
cotinine were made with the mass spectrometers based off mass charge
ratios and the associated retention times, 10.05 min for nicotine
and 12.69 min for cotinine.
Biological sample collection and
preparation
Rats were killed by decapitation and
blood was collected in 2 ml tubes and centrifuged for 15 minutes at
10,000 rpm. The serum was collected and stored at -20 oC
until analysis.
Sample preparation
Serum was collected from each rat and
approximately 2 ml were reserved to be prepared as spiked and
unspiked samples for GC-MS analyses. In spiked samples, 1 ml of
serum was spiked with nicotine and/or cotinine standards for a 500
ppb dilution. Standards were not added to 1 ml unspiked samples. A
solvent solution was made using 1 ml of dichloromethane and 1 ml of
1:1 petroleum ether: ethyl ether. A solution was made by combining a
2:1 ratio of solvent to serum and samples were shaken and allowed to
settle at room temperature. The clear organic layer was collected,
put into a new test tube and evaporated under a nitrogen stream at
room temperature. One ml of methanol was added to the test tube to
suspend the nicotine. The mixture was then transferred to an amber
vial for GC-MS analysis.
RESULTS
Intake
Consumption of the two nicotine
solutions by sex is plotted in Figure 1 as a mean intake score for
the 23 hours preceding blood collection. As evident from these data,
male and female pairs ingested both the 5
mg/ml and the 8
mg/ml nicotine solutions. Male pairs (N = 7) drank similar amounts of the
5 mg/ml
and 8 mg/ml nicotine
solutions (Ms = 23.75 and 21.51), but female pairs (N = 12)
drank less of the 5
mg/ml and more of the
8 mg/ml
nicotine solution (Ms = 17.65 and 24.44). Intake data were
analyzed as a series of ANOVAs with the software program SPSS. The
amount of nicotine solution consumed by pairs was analyzed
statistically with solution (5
mg/ml vs. 8 mg/ml)
as a within subjects factor and sex (male vs. female) as a between
subjects factor but this analysis revealed no significant solution x
sex interactions, F(1, 17) = 1.33, p = 0.26, nor main
effects for sex or solution F(1, 17) = .22 and .34, ns.
Figure 2 illustrates intake data for 10 individually housed rats (6
males and 4 females), an arrangement that allowed for description of
nicotine ingestion by body weight. Once corrected for body weight,
solution x sex interactions were not significant and there was not a
main effect for sex, F(1, 8) = 2.47 and 1.11, ns, but this
analysis exposed a main effect for solution F(1, 8) = 5.44,
p = .048. Adjustments for body weight revealed subjects drank
significantly more of the 8
mg/ml nicotine solution compared to the 5
mg/ml solution
(Ms = .40 and .16). Also depicted in Figure 2 is the enormous
amount of variability in the amounts of the two nicotine solutions
ingested by both male and female rats, an indication that some rats
were very high and others very low consumers of the nicotine
solutions.
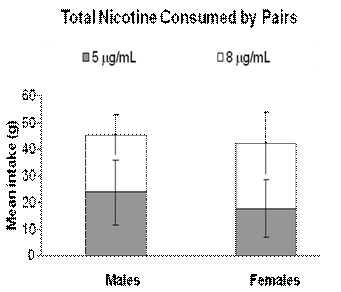
Fig 1.
Mean consumption ± standard error of two nicotine solutions, 5
mg/ml
and 8
mg/ml
by male and female rats housed in pairs and presented with 2 bottles
of each nicotine solution and 2 bottles of water in the home cage.
The male group contained 20 rats and the female group contained 28
rats. Bars reflect mean consumption measured in grams of nicotine
solution during the 23 hours prior to termination.
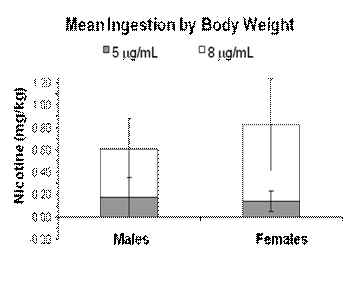
Fig 2.
Mean ingestion ± standard deviation of 5
mg/ml
and 8
mg/ml
nicotine solutions by male (N = 6) and female (N = 4) rats housed
individually. Bars reflect mean nicotine consumption measured in
milligrams per kilogram body weight during the 23 hours prior to
termination.
Gas Chromatography-Mass Spectrometry
Spiked and unspiked serum samples
from 41 of the 48 rats were prepared and analyzed by GC-MS. Reasons
for the exclusion of serum from 7 rats included low serum collection
and in one case mislabeling of the vials so that proper
identification of two subjects was not possible. Nicotine was found
in 28 of 41 (68. 29%) spiked samples and 21 of 40 (52.50%) unspiked
samples, with wide variations in peak areas on chromatograms, an
indication of variations in serum concentration. GC-MS results
indicated the nicotine retention time was 10.05 ± 0.06 min in spiked
and 10.06 ± 0.07 mins in unspiked samples. Detection of cotinine was
less successful as it was found in only 18 out of 41 (43.90%) spiked
and 2 out of 40 (5.00%) unspiked samples. Cotinine retention time
was 12.69 ± 0.08 min and 12.61 ± 0.001 min in spiked and unspiked
samples, respectively. See Figure 3 for the chromotagraphic
separation of nicotine and cotinine in serum samples from one of the
subjects in which cotinine was detected. Figure 4 depicts nicotine
peaks in spiked and unspiked serum samples from the same subject.


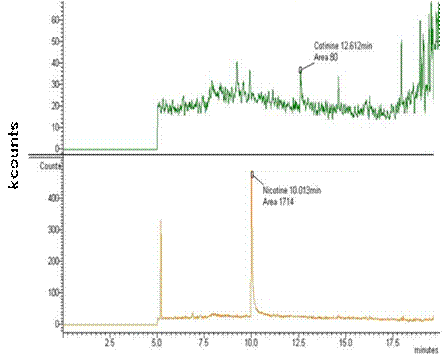
Fig 3.
GC-MS chromatograms showing cotinine (A) and nicotine (B) peaks in
unspiked serum samples from a male rat following oral exposure to
nicotine. The cotinine retention time was 12. 612 minutes, whereas
the nicotine retention time was 10.013 minutes in this subject. Note
differences in the y-axes where peaks reflect kilocounts, or
relative ion counts recorded by instrument software.


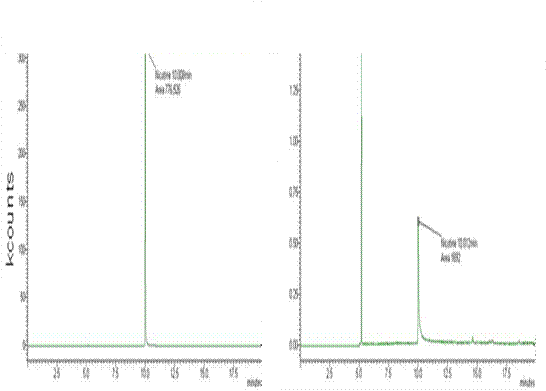
Fig 4.
GC-MS chromatograms revealing nicotine peaks in spiked (A) and
unspiked (B) serum samples from the same female subject following
oral exposure to nicotine. Nicotine retention times were 10.008
(spiked) and 10.012 (unspiked) minutes. The identity of the first
peak in the panel b is unknown. Note differences in the y-axes where
peaks reflect kilo counts, or relative ion counts recorded by
instrument software.
DISCUSSION
Alternative routes of nicotine
delivery, including oral administration, hold promise as aides to
smoking cessation in humans (Martinet, Bohadana, & Fagerström, 2006,
2007; Rose, Herskovic, Trilling, & Jarvik, 1985; Schneider, Jarvik,
& Forsythe, 1984) and these results offer important contributions to
this field. First, they demonstrate that both male and female rats
will readily consume higher concentrations of oral nicotine than
previously reported. Previous studies showed rats tend to consume
relatively small amounts of nicotine in concentrations exceeding 1
mg/ml (Flynn, Webster,
& Ksir, 1989) and modifications such as water deprivation (LeHouezec,
Martin, Cohen, & Molimard, 1989; Lang, Latiff, McQueen, & Singer,
1977), food deprivation (Lang et al.), or sweetening of nicotine
solutions (Smith & Roberts, 1995) have been used to encourage
consumption. Using a nicotine bitartrate solution of 3
mg/ml, we reported
increased consumption by adolescent female rats with no manipulation
other than increasing the availability of nicotine, a result deemed
the multiple bottle effect (Biondolillo & Pearce, 2007). The current
study is the first to report consumption of a 5mg/ml
or 8 mg/ml nicotine
freebase solution in both male and female rats. Considering the
consumption levels reported here, perhaps the threshold for nicotine
tolerance/avoidance has not yet been reached, at least not with the
approach provided by the multiple bottle procedure.
Second, previous published studies
both in our lab (Boyett et al., 2007) and others (Dadmarz & Vogel,
2003) have examined individual differences in intake of oral
nicotine and report high, moderate and low consumers. In unpublished
studies, we have also consistently observed sex differences in
nicotine consumption, with females drinking more nicotine when
adjusted for body weight. Similar findings were reported in
periadolescent mice (Klein, Stine, Vandenbergh, Whetzel, & Kamens,
2004) where sex differences in nicotine consumption, but not serum
cotinine levels, suggested differences in nicotine pharmacokinetics.
Klein and colleagues contended that female mice may more rapidly
metabolize nicotine or perhaps eliminate cotinine more slowly
compared to male mice. Although sex differences in the consumption
data were not observed in our study, this may be due to the small
number of subjects as the observed power on these analyses was
considerably low, falling under 30% for analyses on the solution x
sex interaction effects and the main effect of sex. We contend that
increasing the number of subjects and extending the application of
this method in future studies will offer a comparative tool to
understand the influences on voluntary nicotine use as individual,
sex, and pharmacokinetic differences are also observed in human
tobacco users (Benowitz & Jacob, 1997; Shiffman, 1989, 1991).
Third, these results provide evidence
that despite the oral route using the relatively slow process of
gastric absorption and first-pass metabolism, systemic absorption of
nicotine may result in pharmacologically detectable amounts when
delivered orally, and at least in certain individuals, nicotine
remains available in the bloodstream for up to 2 hours following the
last exposure.
Although the techniques need to be
refined, our study is the first to report determination of nicotine
in serum by GC-MS in rats orally exposed to this drug.
We adapted the methods from other reported
protocols on nicotine delivered via other routes of administration (Cognard
& Staub, 2003; Elobeid, Chai, Clarke, Hannigan, & Russ, 2005;
Elobeid, 2006; Man, et al., 2006) yet additional modifications are
needed. For instance, glassware cleaning procedures may need
to be improved as loss of nicotine by adsorption on glassware has
been reported (Davis, 1986; Teeuwen, Aalders, & Van Rossum, 1989).
Further, when conducting chemical analyses on biological samples, it
is rare to get 100% yield, and such high percentage outcomes often
reflect other problems; although in regard to detection in spiked
samples the aim is to get 90%, a yield considered standard in
chemometric outcomes, this goal may be unattainable under certain
conditions. Inherent variability in serum sample analysis exists in
terms of the performance of the sample within the injector and the
loading of the column with volatiles derived from the sample (Robyn
Hannigan, personal communication). Also, there were times during the
project when the GC or MS did not operate as expected and concerns
of the autosampler no longer injecting sample were considered, as
were the age of the samples and decay of nicotine or cotinine when
samples were prepared but the analyses delayed. Although it is
unclear why nicotine and cotinine were not detected in a higher
percentage of the spiked samples, we anticipate that as we refine
these procedures in subsequent studies, either the detection of
nicotine and cotinine will be more consistent, or an explanation for
the variability will be discovered.
We hope to improve this method in
order to routinely detect nicotine and cotinine in serum and brain
samples. Such information may help us to better understand nicotine
dependence by addressing concerns about both the speed with which
nicotine becomes available and the amount of nicotine ultimately
available to the central nervous system. The next logical step is to
examine particular brain regions for nicotine, nicotine metabolites,
or changes in neurotransmitters or neurotransmitter receptors
following exposure.
Acknowledgments
The authors thank Dr. Kris
Biondolillo for supplying animals and laboratory assistance, Dr.
Robyn Hannigan for advisory and technical support, Mr. Anthony
McMickle for animal care, student contributors Nicole Henderson,
Katherine Lisko, Tamarah McGaughey, Tori Melvin, Hannah Pendergraff,
and Cara Duch, and the anonymous reviewers for their helpful
comments. This study was funded in part by the Arkansas Biosciences
Institute of Arkansas State University, Jonesboro.
REFERENCES
Benkirane, S., Nicolas,
A., Galteau, M. M., & Siest, G. (1991).
Highly sensitive immuno-assays for
the determination of cotinine in serum and saliva: Comparison
between RIA and an avidin-biotin ELISA.
European Journal of Clinical Chemistry
and Clinical Biochemistry, 29(6),
405-410.
Benowitz, N. L., & Jacob, P. III.
(1997). Individual differences in nicotine kinetics and metabolism
in humans. NIDA Research Monograph, 173, 48-64.
Besson, M.,
Granon, S., Mameli-Engvall, M., Cloëz-Tayarani, I., Maubourguet, N.,
Cormier, A., et al. (2007). Long-term effects of chronic nicotine
exposure on brain nicotinic receptors.
Proceedings of the National Academy of Sciences of the United States
of America, 104(19),
8155-8160.
Biondolillo, K. D., & Pearce, A. R.
(2007). Availability influences initial and continued ingestion of
nicotine by adolescent female rats. Neuropsychobiology, 55,
73-80.
Biondolillo, K., Pearce, A. R.,
Louder, M. C., & McMickle, A. (under review). Solution concentration
influences voluntary consumption of nicotine under multiple bottle
conditions.
Bolliger, C. T., Zellweger, J.-P.,
Danielsson, T., Biljon, X., Robidou, A., Westin, A., et al. (2000).
Smoking reduction with oral nicotine inhalers: Double blind,
randomized clinical trial of efficacy and safety. British Medical
Journal, 321(7257),
329–333.
Boyett, K., Pearce, A. R., &
Biondolillo, K. D. (2007). Individual differences in voluntary
self-administration of oral nicotine in female rats. Psi Chi
Journal of Undergraduate Research, 12, 162-169.
Cognard, E., & Staub, C. (2003).
Determination of nicotine and its major metabolite cotinine in
plasma or serum by gas chromatography-mass spectrometry using
ion-trap detection. Clinical Chemistry Laboratory Methods,
41(12), 1599-1607.
Dadmarz, M., & Vogel, W. H. (2003).
Individual self-administration of nicotine by rats. Pharmacology,
Biochemistry, and Behavior, 76(3-4),
425-432.
Davis, R. A. (1986). The determination
of nicotine and cotinine in plasma. Journal of Chromatographic
Science, 24, 134-141.
Elobeid, M. (2006). Speciation
analysis of metals in tobacco smoke using GC-ICP-MS. Unpublished
doctoral dissertation, Arkansas State University.
Elobeid, M., Chai, Y., Clarke, D.,
Hannigan, R., & Russ, J. (2005). Speciation analysis with GC-ICP-MS:
Organometal detection in tobacco smoke. In, G. Holland and D.
Bandura (eds.), Plasma Source Mass Spectrometry: Current Trends
and Future Developments. Royal Society of Chemistry, London UK.
80-88.
Eramo, S., Tassi, C.,
Negri, P., Manta, M., Fraschini, M., & Pedetta, F. (2000).
ELISA analysis of salivary cotinine in
smokers. Minerva Stomatologica, 49(4),
163-168.
Flynn,
F. W., Webster, M., & Ksir, C. (1989). Chronic voluntary nicotine
drinking enhances nicotine palatability in rats.
Behavioral Neuroscience, 103(2),
356-364.
Gutkin, B. S, Dehaene, S., &
Changeux, J. P. (2006). A neurocomputational hypothesis for nicotine
addiction. Proceedings of the National
Academy of Sciences of the United States of America, 134(4),
1106-1111.
Heinrich-Ramm, R.,
Wegner, R., Garde, A. H., & Baur, X. (2002). Cotinine excretion
(tobacco smoke biomarker) of smokers and nonsmokers: Comparison of
GC/MS and RIA results. International Journal of Hygiene and
Environmental Health, 205(6), 493-499.
Hieda,
Y., Keyler, D. E., Ennifar, S., Fattom, A., & Pentel, P. R. (2000).
Vaccination against nicotine during continued nicotine
administration in rats: Immunogenicity of the vaccine and effects on
nicotine distribution to brain. International Journal of
Immunopharmacology, 22(10), 809-819.
Klein, L. C., Stine, M. M.,
Vandenbergh, D. J., Whetzel, C. A., Kamens, H. M. (2004). Sex
differences in voluntary oral nicotine consumption by adolescent
mice: A dose-response experiment. Pharmacology, Biochemistry and
Behavior, 78, 13-25.
LeHouezec, J., Martin, C., Cohen C., &
Molimard, R. (1989). Failure of behavioral dependence induction and
oral bioavailability in rats. Physiology & Behavior, 45,
103-108.
Jacob, P. III., Wu, S., Yu, L., &
Benowitz, N. L. (2000). Simultaneous determination of mecamylamine,
nicotine, and cotinine in plasma by gas chromatography-mass
spectrometry. Journal of Pharmaceutical and Biomedical Analysis,
23(4), 653-661.
Jung, B. H., Chung, B. C., Chung, S.
J., Lee, M.-H., & Shim, S. K. (1999). Simultaneous GC–MS
determination of nicotine and cotinine in plasma for the
pharmacokinetic characterization of nicotine in rats. Journal of
Pharmaceutical and Biomedical Analysis, 20(1-2), 195-202.
Lang, W. J., Latiff, A. A., McQueen,
A., & Singer, G. (1977). Self administration of nicotine with and
without a food delivery schedule. Pharmacology, Biochemistry and
Behavior, 7, 65-70.
Langone, J. J., Cook,
G., Bjercke, R. J., & Lifschitz, M. H. (1988).
Monoclonal antibody ELISA for cotinine
in saliva and urine of active and passive smokers. Journal of
Immunological Methods, 114(1-2), 73-78.
Man, C. N., Gam, L.-H.,
Ismail, S., Lajis, R., & Awang, R. (2006).
Simple, rapid and sensitive assay
method for simultaneous quantification of urinary nicotine and
cotinine using gas chromatography–mass spectrometry. Journal of
Chromatography B, 844(2), 322-227.
Martinet, Y., Bohadana, A., &
Fagerström, K. (2006). Would alternate tobacco products use be
better than smoking? Lung Cancer, 53, 1-4.
Martinet, Y., Bohadana, A., &
Fagerström, K. (2007). Introducing oral tobacco for tobacco harm
reduction: What are the main obstacles? Harm Reduction Journal,
4, 17.
Riah, O.,
Courriere, P., Dousset, J.-D., Todeschi, N., & Labat, C. (1998).
Nicotine is more efficient than cotinine at passing the
blood–brain barrier in rats. Cellular and Molecular Neurobiology,
18(3), 311-318.
Rose, J. E, Herskovic, J. E.,
Trilling,Y., & Jarvik, M. E. (1985).
Transdermal nicotine reduces cigarette
craving and nicotine preference. Clinical Pharmacology and
Therapeutics, 38(4), 450-456.
Schneider, N. G., Jarvik, M. E., &
Forsythe, A. B. (1984).
Nicotine vs. placebo gum in the alleviation of withdrawal during
smoking cessation. Addictive Behavior, 9(2),
149-156.
Sepkovic, D. W.,
& Haley, N. J. (1985). Biomedical applications of cotinine
quantification in smoking related research. American Journal of
Public Health, 75(6),
663-665.
Shiffman, S. (1989).
Tobacco “chippers” —individual differences in tobacco dependence.
Psychopharmacology, 97(4), 539-547.
Shiffman, S. (1991).
Refining models of dependence: Variations across persons and
situations. The British Journal of Addiction, 86(5), 611–615.
Smith, A., & Roberts,
D. C. (1995). Oral self-administration of sweetened nicotine
solutions by rats. Psychopharmacology (Berlin), 120,
341-346.
Spear, L. (2000).
Modeling adolescent development and alcohol use in animals.
Alcohol Research & Health, 24(2), 115-123.
Stolerman, I. P., &
Kumar, R. (1972). Regulation of drug and water intake in rats
dependent on morphine. Psychopharmacologia, 26, 19-28.
Swan, G. E., Habina, K., Means, B.,
Jobe, J. B., & Esposito, J. L. (1993).
Saliva cotinine and recent smoking – evidence
for a nonlinear relationship. Public
Health Reports, 108(6), 779-783.
Teeuwen, H. W. A., Aalders, R. J. W.,
& Van Rossum, J. M. (1989). Simultaneous estimation of nicotine and
cotinine levels in biological fluids using high-resolution
capillary-column gas chromatography combined with solid phase
extraction workup. Molecular Biology Reports, 13, 165-175.
Tyrpien, K.,
Wielkoszyski, T., Janoszka, B., Dobosz, C., Bodzek, D., & Steplewski,
Z. (2000). Application of liquid separation techniques to the
determination of the main urinary nicotine metabolites. Journal
of Chromatography A, 870(1-2), 29-38.
Wall, M. A.,
Johnson, J., Jacob, P., & Benowitz, N. L. (1998). Cotinine in the
serum, saliva, and urine of nonsmokers, passive smokers, and active
smokers.
American Journal of Public Health, 78(6),
699-701.
Warnakulasuriya, S., Sutherland, G., &
Scully, C. (2005). Tobacco, oral cancer, and treatment of
dependence. Oral Oncology, 41(3), 244-260.
Ziegler, U. E., Kauczok,
J., Dietz, U. A., Reith, H. B., & Schmidt,
K. (2000). Clinical correlation between
the consumption of nicotine and cotinine concentrations in urine and
serum by competitive enzyme-linked immunosorbent assay.
International Journal of Experimental and Clinical Pharmacology, 72(4),
254-259.
Accepted for
publication: 10 December 2008
|