Mist reactors: principles, comparison of various
systems, and case studies
Pamela
Weathers1,2, Chunzhao Liu1,3,
Melissa Towler2, and Barbara Wyslouzil4
1
Arkansas State University, Jonesboro, AR; 2 Worcester
Polytechnic Institute, Worcester, MA;
3
National Key Laboratory of Biochemical Engineering, Beijing,
China; 4 The Ohio State University, Columbus, OH
*Corresponding author; email:
weathers@wpi.edu
Keywords:
Artemisia annua, scale-up, gas-phase reactors
ABSTRACT
Growing
hairy roots in bioreactors has proven challenging. Here we
summarize the recent work using a novel bioreactor, the mist
reactor, for the culture of hairy roots from many plant species.
Compared to most liquid-phase bioreactors, this gas-phase
bioreactor offers a completely different environment for growing
roots. Design, modeling, and some of the unique engineering
aspects inherent to mist reactors are explained along with some
of the biological responses of roots when grown in mists
compared to liquids. Prospects for future development of this
technology are also summarized.
INTRODUCTION
Hairy roots
can produce novel compounds not found in the whole plant or in
untransformed roots (Flores and Medina-Bolivar, 1995; Wysokinska
and Chmiel, 1997; Flores et al., 1998), often at levels
exceeding those found in the parent plant (Wysokinska and
Chmiel, 1997; Wysokinska and Rozga, 1998; Rao and Ravishankar,
2002). They also offer significant advantages for the production
of engineered proteins (Guillon et al., 2006; Zhang et al.,
2005; Shanks and Morgan, 1999). Although there are some reports
of co-cultured differentiated tissues (e.g. shoots plus roots)
being used to produce secondary metabolites (Mahagamasekera and
Doran, 1998; Subroto et al., 1996), most efforts are focused on
hairy roots.
Several
bottlenecks, however, hamper the routine production of
compounds, especially secondary metabolites, from hairy roots.
The two main challenges are: (1) for many products the
biochemical pathways and their regulation are not understood;
and (2) although progress has been made, cost effective
scaleable production systems are not yet well developed.
Developing an inexpensive scaleable reactor for hairy roots
would address the latter problem, enabling large-scale culture
and the production of many important and valuable chemicals
(Guillon et al., 2006). Recent political challenges to the
release of transgenic plants further warrant the use of
controlled production schemes including the use of low cost
bioreactors (Guillon et al., 2006).
Our
research has focused on gas-phase (mist) reactors, because this
environment reduces the gas-exchange limitations and the high
shear conditions normally found in liquid-phase reactors. Unlike
growth in liquid systems, roots grown in mist reactors are not
oxygen limited (Weathers et al., 1999) even at high bed
densities (Kim, 2001), and the production of secondary
metabolites is often greater in mist reactors than in liquid
phase reactors (Kim et al., 2001; Bais et al., 2002). High
biomass density is required for a reactor system to be
economically viable, and the maximum root tissue concentration
that can be achieved in a bioreactor depends on the efficient
mass transfer of oxygen and other nutrients into the dense root
matrix (Curtis, 2000). A point in favor of gas phase reactors is
that roots are more able to compensate for poor liquid
dispersion than for poor gas dispersion within a reactor
(McKelvey et al., 1993). A recent comparison by Suresh et al.
(2005), also verified that a mist bioreactor is superior to
other reactor designs for hairy root growth. Finally,
establishing a hairy root culture on solid medium does not
guarantee that they will grow well in liquid medium (Hallard et
al., 1997), so gas-phase reactors may be the only option.
Together these results provide compelling reasons to study mist
reactors.
Recent
reviews have added new perspectives regarding the prospects and
challenges of producing secondary metabolites from hairy roots
in bioreactors (Kim et al., 2002; Towler et al., 2006; Weathers
et al., 2006). These reactors, however, have not yet been scaled
to commercially useful sizes. The key scale-up concerns for
hairy root bioreactors include the following: the flow and
geometric configurations should be scale independent; high
growth rates must be maintained with no limitations in liquid
and gas (O2) nutrients; the key operating parameters
must be identifiable and measurable so that scale-up can be
achieved easily and economically (Cuello et al., 2003). Here we
provide a review on mist/spray reactors and the efforts to scale
them up.
LIQUID VS. GAS PHASE
REACTORS
O2,
CO2 and C2H4 are the three
gases most important to plants, but all are poorly soluble in
water; their solubility decreases with increasing nutrient
concentration and temperature (Atkinson and Mavituna, 1991;
Geankopolis, 1993), and oxygen is about 25 times less soluble
than CO2. To ensure rapid growth of roots, high
levels of oxygen are required while excessive CO2
accumulation must be prevented as the level of respiration
increases with increasing root biomass. Liquids therefore limit
the amount of gas available to growing roots.
Good gas
transfer into liquid media can be obtained by either dispersing
the liquid into the gas or by dispersing the gas into the
liquid. In liquid-based reactors, gas is dispersed into the
liquid phase, while in a mist reactor liquid is dispersed into
the gas phase. Liquid dispersion can be achieved using a number
of different methods including a spinning disk, a compressed gas
atomizer, or an ultrasonic droplet generator. The spinning disk
produces a wide range of droplet sizes and is mainly used in
whole plant aeroponic systems (Weathers and Zobel, 1992).
Smaller droplets have a larger surface to volume ratio that
facilitates gas transport into liquid. Compared to the spinning
disk, compressed gas atomizers can produce rather uniform
amounts of very small droplets thereby ensuring good gas
transfer into the liquid and thus, to the roots. Unfortunately
to obtain small droplets, the nozzle orifice must be small and,
as a result, it clogs easily.
Ultrasonically produced mists have proved to be the most
effective method of generating nutrient aerosols for in vitro
cultures and have, thus, been the focus of most mist reactor
studies with hairy roots. Earlier mist reactors used a submerged
ultrasonic transducer producing sound waves in the 1.7 MHz
range. Although the mechanism of energy transmission of this
type of misting system has been discussed in depth elsewhere
(Weathers and Wyslouzil, 2007), the transducer energy can affect
the mean droplet diameter, Dn, which depends on the
density ρ the surface tension of the liquid σ and the
transducer frequency Ʋ as Dn = 0.34(8πσ/ρ Ʋ2)(1/3)
(Lang, 1962). At higher frequencies Dn also depends
on the power density at the liquid surface (Tarr et al., 1991).
One of the
most important factors in a mist or spray reactor is the droplet
size, and Table 1 summarizes the three broad categories
generally used to classify droplets dispersed in a gas. Smaller
droplets have several advantages including long settling times
that ease transport and distribution of the dispersed nutrient
solution and enhance the ability of the small droplets to
penetrate dense tissue cultures and more evenly distributing
liquid nutrients, as well as large surface to volume ratios that
ensure good mass transfer of gases between the gas and liquid
phases enhancing gas transfer to the root tissue. If the droplet
size is too small, however, inadequate medium will be supplied
to the roots. Excessively large droplets, on the other hand,
will impede adequate gas transfer into the roots. Although the
optimum droplet size is not known, and has not been studied
other than by measuring droplet deposition in dense beds of
hairy roots (Wyslouzil et al., 1997), Weathers and Zobel (1992)
suggested that, for roots, the minimum acceptable droplet size
is probably about 1 mm.
Table 1.
Droplet size ranges for mists, fogs and sprays. (Perry and
Green, 1997; Weathers and Zobel, 1992; Whipple, 1995).
Droplet type |
Droplet diameter
(mm) |
Droplet forming system |
Droplet diameter
(mm) |
Mist |
0.01-10 |
ultrasonic systems |
1-35
Dv ~ 7-10 is typical |
Fog |
1-100 |
compressed gas atomizers |
1-100 |
Spray |
10-10,000 |
spray nozzles |
>100 |
A BASIC
MIST REACTOR
A basic
mist bioreactor (Figure 1) is rather simple. It is comprised of
a mist generating system, a culture medium reservoir, a liquid
pump (usually peristaltic), a sterile filtered air supply, a gas
flow meter, a timer to regulate misting time, and a culture
chamber. Most mist reactors also require a trellis or other
means of support for suspending roots in the growth chamber.
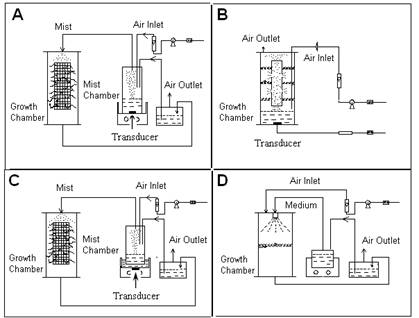
Figure. 1
Mist bioreactor designs for hairy root cultures: A) the
ultrasonic mist generator and growth chamber are in separate
vessels; the ultrasonic transducer (arrow) is inside the mist
generator sump; B) the ultrasonic mist generator and the growth
chamber are in the same vessel; C) the ultrasonic transducer
(arrow) is separated from the growth chamber sump by an acoustic
window which is also the base of the mist generator; D) trickle
bed bioreactor.
Table 2.
Summary of hairy root studies in mist or spray reactors.
Plant Genus |
Major Results |
System Used |
Working Vol. (L) |
Reference |
Artemisia
|
growth comparable to flasks and
plates |
acoustic window1 mist
reactor |
na |
Buer et al., 1996 |
modified inner-loop reactor growth
comparable to flasks |
submerged ultrasonics |
2.3 |
Liu et al., 1999 |
no O2 limitation, but 50%
less biomass than liquid systems |
acoustic window2 mist
reactor |
1.5 |
Weathers et al., 1999 |
altered branching rate versus flasks
– single root studies |
acoustic window2 mist
reactor |
na |
Wyslouzil et al., 2000 |
3x higher artemisinin content than
bubble column |
acoustic window2 mist
reactor |
1.5 |
Kim et al., 2001 |
growth comparable to bubble column |
acoustic window2 mist
reactor |
1.5 |
Kim et al., 2002a |
altered terpenoid gene expression
versus flasks |
acoustic window2 mist
reactor |
1.5 |
Souret et al., 2003 |
|
in 5% sucrose growth exceeded that in
shake flasks |
acoustic window2 small
mist reactor |
0.035 |
Towler et al., 2007 |
Beta |
growth comparable to flasks |
submerged ultrasonics |
1 |
Weathers et al., 1989 |
Carthamus |
growth comparable to flasks; 15%
faster than airlift reactor |
submerged ultrasonics |
1 |
DiIorio et al., 1992 |
Cichorium |
higher biomass and esculin content
than bubble column |
acoustic window3 mist
reactor |
1.75 |
Bais et al., 2002 |
Datura
|
1.6x lower doubling time than
submerged cultures |
droplet reactor |
2 |
Wilson et al., 1990 |
successful large-scale
culture |
hybrid submerged/ droplet reactor |
500 |
Wilson, 1997 |
Fragaria |
biomass yield higher than droplet
bioreactor |
mist reactor |
1 |
Nuutila et al., 1997 |
Hyoscyamus |
growth comparable to shake flask |
spray reactor |
0.30 |
McKelvey et al., 1993 |
Nicotiana |
50% lower doubling time than flasks |
spray reactor |
0.50 |
Whitney, 1990, 1992 |
Panax |
altered ginsenoside pattern versus
native rhizome |
spray reactor |
1 |
Palazon et al., 2003 |
Stizolobium
|
intermittent feeding promoted L-DOPA
production |
mist trickling reactor |
3 |
Huang et al., 2004 |
higher L-DOPA productivity with
higher inoculum density |
mist trickling reactor |
9 |
Huang and Chou, 2006 |
root branching increased biomass and
L-DOPA content |
mesh hindrance mist trickling reactor |
5 |
Sung and Huang, 2005 |
Tagetes |
growth and thiophene content
comparable to shake flask; higher than bubble
column, nutrient sprinkle |
acoustic window3 mist
reactor |
0.30 |
Suresh et al., 2005 |
1, Conap’s
EN6, 2, Teflon, 3, polycarbonate, na, not applicable.
It is
possible that the size of such a support structure can be
minimized if it is coated with poly-L-lysine to induce root
attachment (Towler et al., 2003). How these components are
designed and then configured has enabled a variety of mist
reactors to be employed for culturing many different biological
tissues or organisms. For example, mist reactors have been
successfully used to culture animal tissues (Friberg et al.,
1992), to study whole plants (Souret and Weathers, 2000;
Sharaf-Eldin and Weathers, 2006), in micropropagation (Correll
and Weathers, 2001a, 2001b; Correll et al., 2001; Weathers and
Giles, 1988 and 1989), and to culture hairy roots (Table 2).
Figure 1 illustrates some mist reactor designs that have been
employed for hairy root culture.
NUTRIENT
MIST DROPLET DEPOSITION MODEL
In
experiments by Kim et al. (2002), roots grown in a bubble column
reactor consistently yielded more dry mass than roots harvested
from a mist reactor. After comparing the biological demand of
the growing roots in the mist reactor to the level of substrate
(sugar) deposited on the roots as a function of reactor packing
fraction, and the deposition of medium droplets using a mist
deposition model (Wyslouzil et al., 1997), Kim et al. (2002)
suggested that roots at low packing fraction could not capture
enough mist to grow at rates comparable to roots grown in liquid
culture. At higher packing fractions, on the other hand, the
opposite appeared to be true – roots grew more rapidly.
In the mist
deposition model (Wyslouzil et al., 1997), root beds are treated
as if they are fibrous filters. For a fixed droplet size Dp,
the droplet capture efficiency (hB)
of the root bed is given by
hB
= 1-exp[(-4LahC)
/ (DR(1-
a))],
where α is the packing fraction, L is the length
of the root bed, DR is the diameter of the
root and
hC
is the combined capture efficiency due to impaction and
interception (hIMP+INT),
and diffusion (hD),
and these efficiencies are all functions of Dp
(Crawford, 1976; Friedlander 1977). The overall mass deposition
efficiency (hOM)
of the root bed is the product of the root bed efficiency
hB(DPi)
and the mass fraction m(DPi) of mist
particles of diameter DPi, summed over the
aerosol size distribution data:
hOM
= ∑ihB(DPi)
× m(DPi). Finally, the medium captured
by the roots (Vdep, mL d-1) is
expressed as: Vdep = 24w
× QL ×
hOM,
where the factor of 24 converts from hr to d,
w
is the duty cycle (min hr-1), and QL
is the medium flow rate (mL min-1) during the mist
“on” cycle. The model is valid only when the flow rate is low
and the Reynold’s number (Re), based on the root diameter, is <
10 (Wyslouzil et al., 1997).
The medium
required to support the growth of roots (Vreq,,
mL d-1), in turn, depends on the amount of biomass
present, its growth rate
m
(d-1), the apparent biomass yield of the
growth-limiting nutrient YX/S (g DW biomass
per g nutrient consumed) and the concentration of the limiting
nutrient in the medium CS (g L-1).
To maintain a desired growth rate,
m,
Vdep ≥ Vreq. Kim et al.
(2002) suggested that at low packing fractions a lack of
nutrients was likely limiting A. annua hairy root growth
in the mist bioreactor. Since Vdep is a strong
non-linear function of
a,
increasing
a
rapidly increases Vdep thereby supporting a
higher growth rate because the roots are now capturing more
nutrients. Although Vreq also depends on
a,
that relationship is linear. Kim et al. (2002) showed that Vdep
can be greater than Vreq, but depends on the
nutrient concentration, as well as the growth rate and diameter
of the roots.
LAB SCALE
STUDIES
Exploiting
the Mist Deposition Model to Improve Root Growth in Mist
Reactors
The mist
deposition model suggested several ways to improve the growth
rate of roots in a mist reactor beyond that observed by Kim et
al. (2002). First, increasing the initial biomass density
(packing fraction, a) should increase the droplet capture
efficiency of the roots and better satisfy the biological
demand. Second, increasing the total carbon available to the
roots, either by increasing the sugar concentration (CS) of the
medium or by increasing the mist duty cycle (w), should also
increase the growth rate. To test these hypotheses, Towler et
al. (2007) constructed a small mist reactor (0.05 L growth
chamber) and conducted an extensive number of short (6 day) root
growth experiments.
As
illustrated in Figure 2, the measured growth rates of 0.12 d-1
over a 6-day period at initial packing fractions in the small
mist reactor ranging from 0.19-0.39. In the work of Kim et al.
(2002), packing fractions were no more than 0.056 when mist mode
commenced and the growth rate (m)
was ~ 0.07 d-1 (points at
a
<0.1 in Figure 2). The work by Towler et al. (2007), confirmed
that a higher initial inoculum density does positively affect
root growth in mist reactors.
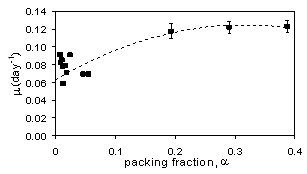
Figure 2.
Hairy root growth vs. packing fraction, α, in mist reactors.
To test the
limits of packing density that can be achieved in a mist
reactor, roots were grown in the small mist reactor from an
initial α of 0.39 for 10.3 days. Although the average
growth rate was only 0.11 d-1, the final α was
0.71, and, as illustrated in Figure 3, the harvested roots
appeared non-necrotic and healthy throughout the dense bed. In
liquid-phase culture such high biomass densities are difficult
to achieve because at tissue concentrations approaching 10-40 g
DW L-1 (a range of α ~ 0.20-0.80) the root bed
resists fluid flow resulting in too high a pressure drop
(Carvalho and Curtis, 1998; Ramakrishnan and Curtis, 2004) with
a liquid flow resistance similar to a column of packed sand
(Carvalho and Curtis, 1998; Nield and Bejan, 1991).
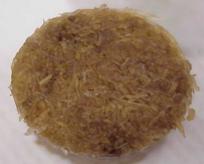
Figure 3.
Roots removed from small mist reactor. Final packing density =
0.71; root bed diameter is 2.7 cm. Upper panel is side view;
lower panel is cross sectional view of roots half way down the
height of the bed.
Besides the
packing density, α, one of the other key components of
the mist deposition model is the nutrient concentration, CS.
To grow at a particular growth rate, µ, the
volume of medium required by the roots, Vreq,
is inversely proportional to CS. Increasing
CS should, therefore, increase µ at fixed
Vdep, or decrease Vreq at
fixed µ. Towler et al. (2007) tested this hypothesis
using the small (0.05 L) mist reactor. A. annua hairy
roots were fed medium containing 3% or 5% sucrose at a standard
mist duty cycle (15 min on, 15 min off). After 6 days, roots
grown with 5% sucrose had significantly more biomass than roots
grown with 3% sucrose (Table 3), while the highest growth rate
occurred at day 4. The growth rate at day 4 with 5% sucrose in
the mist reactor is equivalent to that in shake flasks. Although
we have tried to grow roots in shake flasks at α values
equivalent to what we used in the small mist reactor, there was
little to no growth (Towler, 2005). Taken together these results
corroborate the mist deposition model’s (Wyslouzil et al., 1997)
prediction that at lower root bed packing densities (α ≤ 0.1)
roots cannot capture all of the droplets. Thus, especially in
the early stages of operation (just after inoculation), media
with a higher sucrose concentration must be provided to deliver
adequate levels of carbon to achieve and sustain a high growth.
Although most of the droplets are captured once α reaches ~ 0.2,
maintaining high growth rates in these densebeds also requires
maintaining a high sucrose concentration to feed the dense root
population.
Table 3.
Sugar concentration increases growth in mist bioreactors after 4
and 6 days. Initial DW of inoculum was 8 g FW L-1
(0.52 g DW L-1).
Growth Condition
(working volume) |
DW (g) |
µ (d-1) |
Mist reactor (35 mL) |
4 d |
6 d |
4 d |
6 d |
3% sucrose |
1.11 |
1.22 |
0.16 |
0.12 |
5% sucrose |
1.29 |
1.77 |
0.20 |
0.13 |
Shake flasks (50 mL) |
|
|
|
|
3% sucrose |
0.05 |
0.07 |
0.15 |
0.17 |
5% sucrose |
0.06 |
0.08 |
0.19 |
0.18 |
An
alternate method for providing more nutrients to root cultures
in a mist reactor is to increase the misting duty cycle,w
(min hr-1) which increases the medium flow rate, QL,
(mL min-1), and thus, the total delivery of nutrients
to the roots. The mist duty cycle, however, can significantly
affect the growth rate of roots in a mist bioreactor (Towler et
al., 2006; Liu et al., 1999) with some evidence of species
specificity (DiIorio et al., 1992b). Recent experiments by
Towler et al. (2007) separated the effect of duty cycle from
concurrent changes in the sugar deposition rate. By varying the
mist duty cycle and the sucrose concentration together so that
the average sugar deposition rate was constant, they showed that
alteration of the mist duty cycle had no significant effect on
A. annua root growth. In contrast, when different sugar
concentrations were fed using the same misting cycle, then
growth increased as sugar concentration increased (Towler et
al., 2007). Taken together these studies showed that α
and CS are the two most important factors to
optimize in order to achieve high growth rates of hairy roots in
mist reactors.
Other
Factors Affecting Root Growth in Mist Reactors
Besides
α, CS , and
w
from the mist deposition model, other factors have been observed
to affect root growth in mist reactors including mist flow
orientation, inoculum distribution, gas composition, medium
“conditioning” factors, and medium preparation methods. Mist
reactors that are fed from the top down have the advantage of
co-current down-flow of gas and liquid phases along with gravity
and this facilitates drainage. If mist is provided from the
bottom up, coalescence is greatest on the roots closest to the
mist feed so less mist reaches tissues in the top layers of the
growth chamber (Liu et al., 1999). With increasing root
bed density, the lower regions will likely become waterlogged if
mist is provided upwards. Indeed using upflow mist delivery
(Chatterjee et al., 1997) resulted in root necrosis in A.
annua a condition that was never observed with downflow mist
delivery (Wyslouzil et al., 2000). It is also likely that as the
root bed becomes very dense, the lower sections will accumulate
even more liquid and essentially become submerged.
If the root
inoculum is not evenly distributed within the growth chamber of
a mist reactor, a large percentage of the mist will exit the
chamber without contacting any roots. Furthermore, roots that
capture mist droplets will probably grow rapidly and
subsequently form dense root clusters. Most mist reactors have
been inoculated by first flooding the growth chamber with media,
adding the roots, and then running the reactor as a bubble
column until the roots immobilize onto a trellis.
Like roots
of whole plants, hairy roots also respond to changes in gas
composition with their response varying with plant species.
Besides oxygen most other gas studies have focused mainly on CO2.
Air enriched with CO2 enhanced growth by decreasing
the lag time in some root cultures (30), but not in others
(Wyslouzil et al., 2000; Kim et al., 2002). If CO2 is
observed to stimulate hairy root growth for a species under
study, it is important not to overaerate a root bed in a mist
reactor to avoid purging beneficial gases. Alternatively, the
ability to provide a controlled amount of CO2
enriched air to root beds is one of the design benefits of using
a mist reactor. The effect of changing the combined levels of O2,
CO2, or C2H4 has not, however,
been studied to date.
Reactors
can be operated in a batch mode where coalesced mist culture
medium is collected and recycled back into the reservoir after
exiting the growth chamber. They can also be run in a continuous
mode whereby the culture medium only passes over the tissue once
prior to being discarded, and fresh medium is continuously added
to the reservoir. Although the latter supplies fresh medium and
would be expected to stimulate growth, usually it does not
because roots often prefer “conditioned” medium. Conditioned
medium is produced by growing roots in fresh media for up to 2
weeks (Wyslouzil et al., 2000) during which time they adjust the
pH and distribution of nutrients. Roots also exude growth
stimulating compounds including oligosaccharides (Schroder et
al., 1989), peptides (Matsubayashi et al., 1996), and auxins
(Weathers et al., 2005). Conditioned medium can easily be
introduced into the medium reservoir by filter sterilization.
Most
reactor studies use medium prepared by autoclaving. During this
process however, the sucrose in the medium is partially
hydrolyzed to fructose and glucose, and small amounts of toxic
byproducts are produced (Weathers et al., 2004) resulting in an
undefined medium. Such variations can lead to aberrations in
hairy root growth (Kim et al., 2002) and secondary metabolite
production (Kim et al., 2002; Weathers et al., 2004) in both
liquid and gas-phase culture systems. Use of filter sterilized
medium eliminates such problems.
SCALING UP
MIST/SPRAY REACTORS
Despite
many lab scale studies, there have been few in depth studies on
scaling up mist or spray reactors for hairy root cultures. One
early study at 500 L used a series of hanging hooks to
immobilize Datura roots that were then grown for 40 days
(Wilson, 1997). Productivity, however, was low at 1.3 g DW L-1
d-1 with a final packing density of about 8% (α
= 0.08; FW V-1). Three more recent studies had
more productive growth yields (Williams and Doran, 2000;
Ramakrishnan and Curtis, 2004; Huang and Chou, 2006), and the
key elements of those studies are summarized in Table 4. In
contrast to the fine mists (droplets~10 µm) used by the Weathers
and Liu labs (Table 2), the studies shown in Table 4 used
sprays, with median volume droplet diameters exceeding 50 µm.
Williams and Doran (2000) concluded that their spray reactor was
not effective because as the biomass density of the root bed
increased the large droplets resulted in the root bed becoming
water logged and gas exchange plummeted. Indeed Ramakrishnan and
Curtis et al. (2004) noted that in their spray reactor large
amounts of interstitial liquid accumulated, and they needed to
enrich the aeration gas with oxygen to maintain growth.
Furthermore, the liquid feed rate (L d-1) in these 3
studies often exceeded the volume of the culture vessel further
aggravating liquid accumulation. For example, in a 14 L
vessel Ramakrishnan and Curtis (2004) provided 648 L d-1
of liquid media to a maximum of 752 g FW of roots (Table 4).
They initiated their reactors in a liquid bubble column mode for
~12 d, shifted to trickle bed mode for the next 8-9 d to enhance
aeration, and eventually enriched the air to 37% O2
in order to maintain high growth rates during the last 5 days of
culture. Only then were they able to achieve a final high yield
of root biomass (Ramakrishnan and Curtis, 2004).
Although
the 14 L trickle bed reactor run by Ramakrishnan and Curtis
(2004) reached a final
a
= 0.75
(Table 4), the growth rate was 0.13 d-1 without
oxygen enrichment and 0.21 d-1 with oxygen
enrichment. In contrast their scaled-down (1.6 L) version of
that reactor yielded a higher growth rate of 0.35 - 0.40 d-1
for the first 4 days. Unfortunately, throughout the rest of the
culture period the growth rate steadily declined to < 0.10 d-1.
The small fine mist droplet reactor used by Towler et al. (2007)
was much smaller (0.05 L), yet a biomass density comparable to
that obtained by Ramakrishnan and Curtis (2004) was attained
with an
a
= 0.71
and overall growth rate of 0.11 d-1.
This was,
however, achieved without addition of extra O2 or
other nutrients (Towler et al., 2007). The 1.6 L trickle bed
reactor (Ramakrishnan and Curtis, 2004) had a biomass/medium
ratio about 8´ greater and a bed depth about 5´ deeper than the
small mist reactor of Towler’s, never the less the high packing
density obtained in the small mist reactor substantiates the
importance of droplet size and total liquid throughput in gas
phase reactors. Clearly, however, more equitable performance
comparisons must be made to determine the potential of larger
scale mist reactors.
In mist
reactors, therefore, it is critical to keep droplet diameter
small and liquid throughput must not exceed levels that result
in water logging of the culture. If the latter occurs, then a
mist reactor reverts to a liquid-phase reactor. In the mist
reactor high-density root beds grow faster than low density
beds, because high-density beds capture media droplets more
efficiently (Towler et al., 2007). This counterintuitive
reactor, however, poses a challenge to scale-up because the high
inoculum levels required to create a dense root bed in a mist
reactor quickly become impractical as the reactor volume
increases. Taken together it is clear that gas-phase reactors
must be carefully designed, studied, and operated to achieve the
high growth yields that we (Towler et al., 2007), and others
(Suresh et al., 2005; Ramakrishnan and Curtis, 2004), have
observed.
CONCLUSIONS
AND FUTURE RECOMMENDATIONS
Mist and
other gas-phase reactors clearly offer some significant benefits
for culturing hairy roots, including lack of oxygen stress,
rapid growth, and production of high yields and, sometimes, of
new secondary metabolites. Scaling up these reactors however,
presents some unique challenges. For example, what is the
volumetric limit of a mist reactor? How does root morphology
alter performance of a reactor? Do thick hairless roots grow
more effectively than thin hairy ones? The mist deposition model
(Wyslouzil et al., 1997) suggests that may indeed be the case.
If so, how does one engineer a reactor to accommodate variations
in root morphology? These types of questions along with others
need to be addressed before mist and other gas-phase reactors
become practical.
ACKNOWLEDGEMENTS
The authors
thank the following agencies for funding some of the described
work: DOE P200A50010-95, NSF BES-9414858, USDA 93-38420-8804,
and NIH 1R15 GM069562-01.
Table 4.
Comparisons between three recently scaled-up gas-phase hairy
root reactors.
Reactor parameters/Results |
Huang & Chou (2006) |
Ramakrishnan & Curtis (2004) |
Williams & Doran (2000) |
Plant species |
Stizolobium hassjoo |
Hyoscamus muticus |
Atropa belladonna |
Working vol (L) |
9 |
14 |
4.4 |
Aspect ratio |
2.1 |
6.0 |
1.7 |
Median drop diameter (µm)
1 |
> 50 |
> 150 |
> 50 |
Medium flow (L min-1) |
0.12 |
0.45 |
0.13 |
Misting cycle |
1 hr on:1 hr off |
continuous |
continuous |
Gas flow (vol gas/working vol/ min (vvm)) |
0.022 |
~ 0.7 |
0.27 |
Inoculum (g DW L-1) |
0.15 (est) |
0.2 |
0.18 (est) |
Final yields (g): FW L-1
α
DW L-1 |
83 (est)
0.083
6.95 |
752
0.752
36 |
182 (est)
0.182
11.23 (est) |
µ
(d-1) [run length in days] |
0.20 (est) [16] |
0.21 [25] |
0.16 (est) [24.9]
0.08 (est) [41.8] |
Notes |
countercurrent dual misting |
cocurrent gas/liquid |
cocurrent gas/liquid |
REFERENCES
CITED
Atkinson B,
Mavituna F (1991) Biochemical Engineering and Biotechnology Handbook,
Ed 2. Macmillan, Basingstoke, UK, pp 703-705
Bais HP, Suresh
B, Raghavarao KSMS, Ravishankar GA (2002) Performance of hairy root
cultures of Cichorium intybus L. in bioreactors of different
configurations. In Vitro Cell Dev Biol Plant 38: 573-580
Carvalho EB,
Curtis WR (1998) Characterization of fluid-flow resistance in root
cultures with a convective flow tubular bioreactor. Biotechnol
Bioeng 60:375-384
Chatterjee C,
Correll MJ, Weathers PJ, Wylslouzil BE, Walcerz DB (1997) Simplified
acoustic window mist bioreactor. Biotechnol Techniq 11:155-158
Correll MJ, Wu
Y, Weathers PJ (2001) Controlling hyperhydration of carnations (Dianthus
caryophyllus L.) grown in a mist reactor. Biotechnol Bioeng
74:307-314
Correll MJ,
Weathers PJ (2001) Effects of light CO2, and humidity on
carnation growth, hyperhydration, and cuticular wax development in a
mist reactor. In Vitro Cell Develop Biol Plant 37:405-413
Correll MJ,
Weathers PJ (2001) One-step acclimatization of plantlets using a
mist reactor. Biotechnol Bioeng 73:253-258
Crawford M
(1976) Air Pollution Control Theory. McGraw-Hill, NY, NY, pp 424-433
Cuello JL,
Walker PN, Curtis WR (2003) Design of ebb-and-flow bioreactor (EFBR)
for immobilized “hairy root” cultures: Part I. Preliminary design
models and culture parameters. Transactions ASAE 46:1457-1468
Curtis WR
(1993) Cultivation of roots in bioreactors. Curr Opin Biotechnol
4:205-210
Curtis WR
(2000) Hairy roots, bioreactor growth. In RE Spier, ed, Encyclopedia
of Cell Biotechnology, John Wiley and Sons, NY, NY, pp 827-841
DiIorio AA,
Cheetham RD, Weathers PJ (1992a) Carbon dioxide improves the growth
of hairy roots cultured on solid medium and in nutrient mists. Appl
Microbiol Biotechnol 37:463-467
DiIorio AA,
Cheetham RD, Weathers PJ (1992b) Growth of transformed roots in a
nutrient mist bioreactor: reactor performance and evaluation. Appl
Microbiol Biotechnol 37:457-462
Flores HE,
Medina-Bolivar F (1995) Root culture and plant natural products:
unearthing the hidden half of plant metabolism. Plant Tiss Cult
Biotechnol 1:59-74
Friberg JA,
Weathers PJ, Gibson DG (1992) Culture of amebocytes in a nutrient
mist bioreactor. In Vitro Cell Develop Biol 28A:215-217
Friedlander SK
(1977) Smoke, Dust and Haze: Fundamentals of Aerosol Behavior,
Wiley, NY, NY, p 338
Geankopolis CJ
(1993) Transport Processes and Unit Operations, Ed 3,
Prentice-Hall, Englewood Cliffs, NJ, pp 586-587
Guillon S,
Trémouillaux-Guiller J, Pati PK, Rideau M, Gantet P (2006)
Harnessing the potential of hairy roots: dawn of a new era. Trends
in Biotechnol 24:403-409
Hallard D,
Geerlings A, van der Heijden R, Lopes Cardoso MI, Hoge JHC,
Verpoorte R (1997) Metabolic engineering of terpenoid indole and
quinoline alkaloid biosynthesis in hairy root cultures. In PM Doran,
ed, Hairy Roots: Culture and Applications. Harwood Academic
Publishers, Amsterdam, The Netherlands, pp 43-49
Huang SY, Hung
CH, Chou SN (2004) Innovative strategies for operation of mist
trickling reactors for enhanced hairy root proliferation and
secondary metabolite productivity. Enz Microb Technol 35:22-32
Huang SY, Chou
SN (2006) Elucidation of the effects of nitrogen source on
proliferation of transformed hairy roots and secondary metabolite
productivity in a mist trickling reactor by redox potential
measurement. Enz Microb Technol 38:803-813
Kim YJ (2001)
Assessment of bioreactors for transformed root cultures. PhD thesis,
Worcester Polytechnic Institute, Worcester, MA
Kim Y,
Wyslouzil BE, Weathers PJ (2001) A comparative study of mist and
bubble column reactors in the in vitro production of
artemisinin. Plant Cell Rep 20:451-455
Kim YJ,
Weathers PJ, Wyslouzil BE (2002) Growth of Artemisia annua
hairy roots in liquid and gas-phase reactors. Biotechnol Bioeng
80:454-464
Kim YJ,
Wyslouzil B, Weathers J (2002) Secondary metabolism of hairy roots
in bioreactors. In Vitro Cell Develop Biol Plant 38:1-10
Kim YJ,
Weathers PJ, Wyslouzil BE (2003) Growth dynamics of Artemisia
annua hairy roots in three culture systems. Biotechnol Bioeng
83:428-443
Lang RJ (1962)
Ultrasonic atomization of liquids. J Acoust Soc A 34:6-8
Liu CZ, Wang
YC, Zhao B, Guo C, Ouyang F, Ye HC, Li GF (1999) Development of a
nutrient mist bioreactor for growth of hairy roots. In Vitro Cell
Dev Biol Plant 35:271-274
Matsubayashi Y,
Sakagami Y (1996) Phytosulfokine, sulfated peptides that induce the
proliferation of single mesophyll cells of Asparagus officinalis
L. Proc Natl Acad Sci USA 93:7623-7627
Mahagamasekera,
MGP, Doran, PM (1998) Intergeneric co-culture of genetically
transformed organs for the production of scopolamine. Phytochemistry
47:17-25
McKelvey SA,
Gehrig JA, Holar KA, Curtis WR (1993) Growth of plant root cultures
in liquid- and gas-dispersed reactor environments.
Biotechnol Prog
9:317-322
Nield DA, Bejan
A (1992) Convection in porous media.
Springer-Verlag, NY, NY, pp 408
Nuutila AM,
Lindqvist AS, Kauppinen V (1997) Growth of hairy root cultures of
strawberry (Fragaria x. ananassa Duch.) in three different
types of bioreactors. Biotechnol Techn 11:363-366
Palazon J,
Mallol A, Eibl R, Lettenbauer C, Cusido RM, Pinol MT (2003) Growth
and ginsenoside production in hairy root cultures of Panax
ginseng using a novel bioreactor. Planta Med 69:344-349.
Perry RH, Green
DW (1997) Perry’s Chemical Engineer’s Handbook.
(McGraw-Hill, New York, NY), 7th Edition, pp. 14-62 –
14-69, 14-81 – 14-82.
Ramakrishnan D,
Curtis WR (2004) Trickle-bed root culture bioreactor design and
scale-up: growth, fluid-dynamics, and oxygen mass transfer.
Biotechnol Bioeng 88:248-260
Schroder R,
Gertner F, Steinbrenner B, Knoop B, Beiderbeck R (1989) Viability
factors in plant suspension cultures – some properties. J Plant
Physiol 135:422-427
Sharaf-Eldin M,
Weathers PJ (2006) Movement and containment of microbial
contamination in the nutrient mist bioreactor. In Vitro Cell Develop
Biol Plant 42:553-557
Souret FF,
KimYJ, Wyslouzil BE, Wobbe KK, Weathers PJ (2003) Scale-up of
Artemisia annua L. hairy roots cultures produces complex
patterns of terpenoid gene expression. Biotechnol Bioeng 83:
653-667
Sung LS, Huang
SY (2006) Lateral root bridging as a strategy to enhance L-DOPA
production in Stizolobium hassjoo hairy root cultures by
using a mesh hindrance mist trickling bioreactor. Biotechnol Bioeng
94:441-447
Suresh B, Bais
HP, Raghavarao KSMS, Ravishankar GA, Ghildyal NP (2005) Comparative
evaluation of bioreactor design using Tagetes patula L. hairy
roots as a model system. Proc Biochem 40:1509-1515
Tarr A, Zhu G,
Browner RF (1991) Fundamentals aerosol studies with an ultrasonic
nebuliser. Appl Spectrosc 45:1424-1432
Towler MJ (2005) Effects of inoculum density, carbon concentration,
and feeding scheme on the growth of transformed roots of
Artemisia annua in a modified nutrient mist bioreactor. PhD
thesis,
Biology and Biotechnology Worcester Polytechnic Institute,
Worcester, MA
Towler MJ, Kim
Y, Wyslouzil BE, Correll MJ, Weathers PJ (2006) Design,
development, and applications of mist bioreactors for
micropropagation and hairy root culture. In SD Gupta, Y Ibaraki,
eds, Plant Tissue Culture Engineering, Springer, The Netherlands, pp
119-134
Towler MJ,
Wyslouzil BE, Weathers PJ (2007) Using an aerosol
deposition model to increase hairy root growth in a mist reactor.
Biotechnol Bioeng 96:881-891
Weathers PJ,
Giles KL (1988) Regeneration of plants using nutrient mists. In
Vitro Cell Develop Biol Plant 24:727‑732
Weathers PJ,
Giles K, inventors (1989) Mist cultivation of cells. U.S. Patent
#4,857,464 and other International Patents (European, Israeli,
Australian, Canadian, New Zealand)
Weathers PJ,
Cheetham RD, Giles KL (1988) Dramatic increases in shoot number and
length for Musa, Cordyline, and Nephrolepis using
nutrient mists. Acta Hortic 230:39‑43
Weathers PJ,
Zobel RD (1992) Aeroponics for the culture of organisms, tissues,
and cells. Biotechnol Adv 10:93-115
Weathers PJ,
Bunk G, McCoy M (2005) The effect of phytohormones on growth and
artemisinin production in Artemisia annua hairy roots. In
Vitro Cell Devel Biol Plant 41:47-53
Weathers PJ,
DeJesus-Gonzalez L, Kim YJ, Souret FF, Towler MJ (2004) Alteration
of biomass and artemisinin production in A. annua hairy roots
by media sterilization method and sugars. Plant Cell Rep 23:414-418
Weathers PJ,
Wyslouzil BE, Wobbe KK, Kim YJ, Yigit E (1999) Workshop on
bioreactor technology. The biological response of hairy roots to O2
levels in bioreactors. In Vitro Cell Develop Biol Plant 35:286-289
Weathers PJ,
Wyslouzil BE (2007) Alternate bioreactors: Mist. In R.E. Spier, eds,
Encyclopedia of Cell Technology, Ed 2, John Wiley & Sons, NY, NY, In
press
Weathers PJ,
Zobel RD (1992) Aeroponics for the culture of organisms, tissues,
and cells. Biotechnol Adv 10:93-115
Whipple M
(1995) Deposition of Nutrient Mist onto Hairy Root Cultures,
M.S. Thesis, Chemical Engineering, Worcester Polytechnic Institute,
Worcester, MA
Whitney PJ
(1990) Novel bio-reactors for plant root organ cultures. Abstracts
VII Intl. Cong. Plant Tissue Cell Cult, Amsterdam, The Netherlands;
Abstract C4-19, p 342
Whitney P
(1992) Novel bioreactors for the growth of roots transformed by
Agrobacterium rhizogenes. Enz Microbiol Technol 14:13-17
Williams GRC,
Doran PM (2000) Hairy root culture in a liquid-dispersed bioreactor:
characterization of spatial heterogeneity. Biotechnol Prog 16:391-401
Wilson PDG
Hilton MG Meehan PTH Waspe CR Rhodes MJC (1990) The cultivation of
transformed roots from laboratory to pilot plant. In HJJ Nijkamp,
LHW van der Plas, J van Aartrijk, eds, Progress in Plant Cellular
and Molecular Biology, Kluwer Academic Publishers, Dordrecht, The
Netherlands, pp 700-705
Wilson DG
(1997) The pilot-scale cultivation of transformed roots. In PM
Doran, ed, Hairy Roots: culture and applications, Gordon and
Breach/Harwood Academic, UK, pp 179-190
Wyslouzil BE,
Waterbury RG, Weathers PJ (2000) The growth of single roots of
Artemisia annua in nutrient mist bioreactors. Biotechnol Bioeng
70:143-150
Wyslouzil BE,
Whipple M, Chatterjee C, Walcerz DB, Weathers PJ, Hart DP (1997)
Mist deposition onto hairy root cultures: aerosol modeling and
experiments. Biotechnol Prog 13:185-194
Wysokinska H,
Rozga M (1998) Establishment of transformed root cultures of
Paulownia tomentosa for verbascoside production. J Plant Physiol
152:78-83
Wysokinska H,
Chmiel A (1997) Transformed root cultures for biotechnology. Acta
Biotechnol 2:131-159
Zhang C,
Medina-Bolivar F, Buswell S, Cramer CL (2005) Purification and
stabilization of ricin B from tobacco hairy root culture medium by
aqueous two-phase extraction. J Biotechnol 117:39-48
Accepted for
publication: 7 October 2008
|